Analysis of the Advantages of Greenhouse Semiconductor Lighting Supplement
Analysis of light source advantages Incandescent lamps are common light sources for creating long-term sunlight, but their electrical efficiency is low, and the low ratio of red to far-red light cannot improve stem elongation, so they are gradually being banned from sale and use. Compact fluorescent lamps and HPS are more energy efficient and have a high R:FR ratio. HPS has three types of 400W, 600W and 1000W. Traditional light sources such as HPS cannot launch low-power products, and their applications are limited. 1000W is more popular because fewer such lamps are needed to achieve the same light intensity. In the practice of supplementary lighting in greenhouses, reducing the number of lamps can effectively reduce the degree of natural light blocked by reflectors of lamps. Energy-saving and efficient regulation of natural light requires an intelligent control system to adjust the amount of artificial light according to the intensity of natural light.
In comparison, the photoelectric conversion efficiency of HPS is 30%, while the incandescent lamp is only 6%, and the photoelectric conversion efficiency of LED lamp is 40%. Other electrical energy is converted to heat energy, heating the greenhouse ambient temperature. In fact, it is uneconomical to use electric heating, and in mild weather conditions, high temperature is not good for production, so it is necessary to turn on fans and other equipment to cool down. The HPS spectrum includes the composite light of yellow light, orange light and red light. It needs to add some blue light to have a more effective light quality. Plants require a certain amount of blue light for normal plant development and morphology. In addition, far-red light is also important for morphogenesis, and the ratio between red, blue and far-red light needs to be adjusted.
LED lights or modules need to be screened for light quality, waterproof performance, compactness, and reduced light-shielding surface area. Heat dissipation methods include water-cooled LEDs, passive gas-cooled LEDs, and active gas-cooled LEDs. LED heat dissipation has always been a problem that needs to be seriously solved. HPS heat dissipation and heating can warm plants and increase respiration. In turn, respiration reduces leaf temperature.
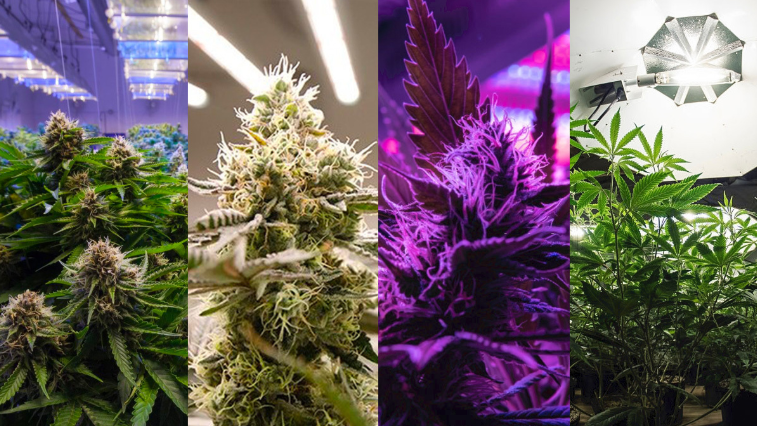
In 2007, some companies in the Netherlands introduced special LED modules for the greenhouse industry. In 2008-2009, some large-scale LED lighting experiments were carried out on roses, tomatoes, bell peppers, cucumbers, and medicinal plants. The results of the experiments were mixed. LEDs have potential for photoperiod regulation and supplementary light application in greenhouses, but there are relatively few studies on horticultural crops, and their application may be limited to special plant production due to high cost (Runkle et al., 2011). LED supplementary lighting in greenhouses is a very promising technology that can effectively capture better plant photosynthesis (red LEDs are higher than HPS), initiate special plant responses or guide plant processes and balance through LED special light quality modulation (Nederhoff, 2010) .
Analysis of lighting technology
Fill light methods include top fill light, interline fill light, multi-layer fill light and other forms. Compared with traditional light sources, the size, shape and power design of LED light source lamps can be freely enlarged, the suspension method is flexible, and the weight is light. It has derived a variety of supplementary light technology modes, which are well adapted to greenhouse planting methods, crop types, and canopy shapes. Diverse practical needs.
Lighting benefit analysis
Plant growth lighting technology is advancing rapidly, providing many options for supplemental lighting in greenhouses. Nelson and Bughee (2014) reported the photosynthetic quantum (400~700nm) efficiency and photon radiation distribution characteristics of 2 kinds of double-sided HPS devices, 5 kinds of mogul-based HPS devices, 10 kinds of LED devices, 3 kinds of cermet lamps and 2 kinds of fluorescent lamps . The 2 most efficient LEDs and the 2 most efficient bifacial HPS devices have almost the same efficiency, between 1. 66 and 1. 7 μmol/J. The efficiency of these four devices is significantly higher than the 1.02μmol/J efficiency of commonly used cermet lamps. 95μmol/J。 The efficiency of the best metal ceramic lamps and fluorescent lamps were 1. 46 and 0. 95μmol / J.
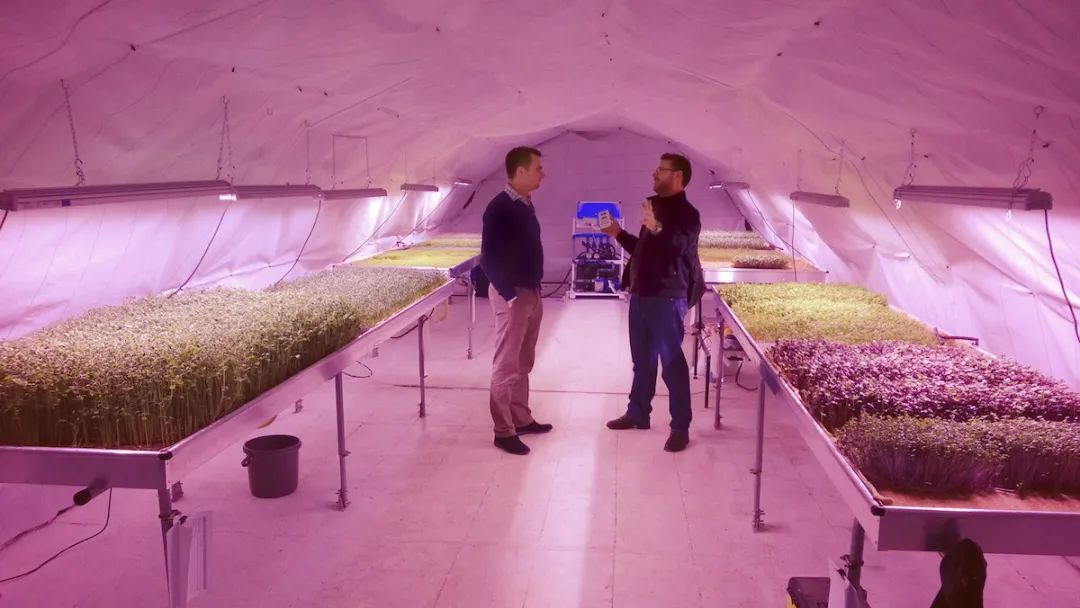
The author calculated the initial investment cost of each light quantum emitted by the device, and clarified that the cost of LED devices is 5 to 10 times that of HPS devices. The 5-year electricity bill plus the cost per mole of photon devices is 2.3 times higher than that of LED devices. In terms of electricity costs, the analysis results show that the long-term maintenance costs are very small. If the production system has a wide gap space, the unique function of the LED device is that it can effectively concentrate the light quantum on a specific part, so that the plant canopy can capture more light quantum. But analysis shows that photon radiation is costly for all lighting fixtures. The lowest lighting system cost can only be achieved when high-efficiency light-emitting devices are combined with efficient canopy photon trapping.
Advances in lighting technology and fixture efficiency have provided many options for supplemental lighting in greenhouses, including many LED fixtures. Great progress has been made in three aspects of lamp composition for high-intensity discharge lamps (HID) [including high-pressure sodium lamps (HPS) and ceramic metal halide lamps (CMI)], including lamps (light bulbs), light sources (reflectors) and ballasts (ballast). The HPS with electronic ballast and double-sided bulb is 1.7 times the HPS of the mogul-based HPS device. The analysis includes two parameters, lamp efficiency, that is, the determination of the number of photosynthetic photons per joule (photons) and the capture efficiency of photosynthetic quantum flow (400-700nm) in the canopy, which is a part of the photons reaching the plant leaves. Plant growth electrical efficiency is measured in the number of photosynthetic photons per joule.
Luminaire electrical efficiency is often expressed in units of human light perception (lumens emitted per watt) or energy efficiency (watts of radiation emitted per watt of electrical input). However, photosynthesis and plant growth are measured in quantum moles of light. Therefore, light efficiency comparisons based on light quantum efficiency should use the unit of photosynthetic quantum quantity produced per joule of energy input. This is even more important for LEDs because the electrically efficient light colors are in the deep red and blue wavelength regions. Red photons have a lower radiant energy capacity allowing more photons to deliver per unit energy input (radiant energy is inversely proportional to wavelength, Planck's equation). Conversely, blue light is 53% more energy efficient than red light (49% and 32%), but blue light is only 9% more photon quantum efficient than red light (1.87/1.72). There are misunderstandings about the effect of light quality on plant growth, and many manufacturers claim that light quality promotes plant growth1 (spectral distribution and ratio of monochromatic light).
The evaluation of the impact of light quality on plant photosynthesis is widely derived from the light quantum yield (YPF) curve, which shows that the red-orange light of 600~660mm is 20%~30% higher than the blue-green and blue light of 400~460nm for photosynthesis. When analyzing light quality based on the YPF curve, HPS performs as well or better than better LED luminaires because it has high photon output around 600nm and lower output in the blue, blue-green, and green light regions.
The spectral curve of quantum abortion was formed on the basis of short-term measurement data under the condition of single leaf and low light intensity (Nelson and Bugbee, 2014). However, YPF curves are drawn from short-term measurements of individual leaves under low-light conditions. The chlorophyll and pigments of chlorophyll have a weak ability to absorb green light (Terashima et al., 2009), but Terashima et al. (2009) pointed out that the photosynthesis efficiency of sunflower leaves driven by green light mixed with strong white light is higher than that of red light. Therefore, green light is often considered ineffective for plant growth, but green light may be effective for plant growth under bright light conditions. High-intensity green LEDs can effectively improve plant growth, especially short-wavelength green light is more effective for plant growth (Johkan et al., 2012).
Over the past 30 years, many long-term studies on whole plants under high light intensity conditions have shown that light quality has a much smaller effect on plant growth rate than light intensity (Cope et al., 2014; Johkan et al., 2012). Light quality, especially blue light, can alter cell and leaf expansion rates (Dougher and Bug-bee, 2004), plant height, plant morphology (Cope and Bug-bee, 2013; Dougher and Bug-bee, 2001) in several plants ; Yorio et al., 2001). But the direct impact of blue light on photosynthesis is minimal. Effects of light quality on whole plant dry and fresh weight generally occur under no or low natural light exposure due to changes in leaf expansion and radiation capture early in growth (Cope et al., 2014).
Based on the number of photosynthetic light quantum moles per joule, the light colors with the highest electrical efficiency of LED light are blue light, red light and cool white light, so LED lamps are generally combined to generate these colors. Other colors of LED light quality can be used to enhance the light quality of specific wavelengths to control certain aspects of plant growth by virtue of monochromatic light properties (Ya2012; Morrow and Tibbitts, 2008). The lack of UV radiation in LED luminaires due to UV-LEDs significantly reduces luminaire efficiency. Sunlight contains UV that accounts for 9% of PPF, and standard electric light sources contain 0.3%~8% of UV radiation. Lack of UV leads to some plant disorders under sunlight conditions (intunmescence, Morrow and Tibbitts, 1988). The lack of far-red radiation (710~740nm) of LED lamps for photosynthetic supplementary light shortens the flowering time of several photoperiod plants (GraigRungle, 2013). Green light (530~580nm), lacking or absent in LED luminaires, is able to penetrate the canopy and be delivered more efficiently to the lower leaves (Kim et al., 2004). That is, the wavelength of each incident light quantum has an impact on the relative photosynthesis of a single leaf under low light intensity (150μmol/㎡).